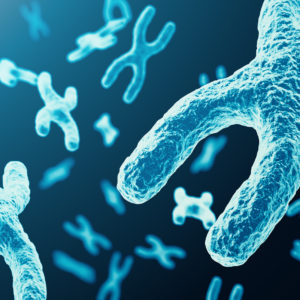
What is Microbiology?
Microbiology is the study of the tiniest forms of organic life (1) - typically, those too small to be seen with the naked eye. The word “microbiology” is, like many scientifically-derived terms, from the Greek language. It consists of the word “mīkros”, translated into English for "small", “bios” which means "life" and “logos” which is “study”. Essentially, microbiology is “small life study”. As microbiology covers many areas, some of which overlap and some that do not, it is subdivided into many separate disciplines; they cover single cell and multicell organisms, viruses, bacteria, algae, fungi, and even complex life such as parasites, and many more. These subdisciplines are listed below.
In recent decades, microbiology has expanded to include practical applications such as biotechnology, and the study of the building blocks of life such as genes and proteins. These are not lifeforms in themselves, but every form of life needs them to live and evolve. There was once also debate over whether viruses could or should be classified as biological life as it was believed they were complex molecules. All microforms of life are vital to the planet's processes and we are still learning just how important an ecological function they provide to the planet's various ecosystems, in keeping environment's “clean” and in helping organic matter decompose or break down as part of the natural cycle of life.
Some of our most important recent discoveries, particularly in medical science (with vaccines being one of the most important breakthroughs of all time) (2), have been the direct result of advances in the research of microorganisms. In the future, it is expected to be at the heart of such disparate areas as environmental remediation, renewable energy, and of course, continue to deliver results in medical science.
Learn more about becoming a microbiologist and environmental microbiology degrees.
A History of Microbiology
The history of microbiology is one of centuries of theoretical science that early thinkers were unable to prove their existence. This would be the case until way into the enlightenment with the discovery of the first microscopes.
Microorganisms: An Early Hypothesis in Antiquity
The credit of the theory of microorganisms goes back some 2,500 years and to the ancient Far East. A follower of the religious teaching of Jainism known as Mahavira postulated the idea that creatures too small for the human eye to see might live in the four major elements of the planet - in the earth, water, air and even in fire (3, 209). He called these “nigodha” and further hypothesized that these microorganisms lived in all living matter in all elements of the universe. However, without modern understanding of evolutionary biology, Mahavira's hypothesis was limited to his religious belief that there was a natural order of lifeforms and that depending on the amount of karma we attain in a previous life, it would dictate which form of life we would come back as in the next (3, p210).
It is unsurprising that we see Hippocrates commenting on this too. As the father of medicine, he hypothesized and theorized about a great many things. Although he could not demonstrate the existence of microbes, he did theorize extensively about their potentially harmful nature. He was observing the greater instances of illnesses in people living in swampy areas (4). This fact was not lost on later Roman writer Marcus Terentius Varro who wrote extensively about microbes in his famous works Three Books of Agriculture. He made several observations, building on Hippocrates work, about the effects of living in swampy areas. Remarkably, he commented that such microbes could enter the body through the nose, mouth and other orifices which, in many cases, later turned out to be true.
The ancient peoples must have had some rudimentary understanding of microbiology even if they could not explain it or explain how it worked. We see it used as an applied science, particularly in biological warfare. Examples exist in both historical texts and literature about using weapons caked with putrefied animal entrails, and human and animal feces, to induce infection in victims (5). Similarly, the Hittites covered their rams with congealed animal remains infected with tularemia.
The Islamic East Preserves Ancient Knowledge
The history of medieval Europe is one of regression and anti-intellectualism. But in the Islamic Middle East, works by Hippocrates and other classical writers taken there during the Greek and Roman periods of conquest, survived, were studied and built upon. Although the world was far from developing a proper scientific understanding of microbiology, and centuries away from developing tools for their discovery (the microscope), several popular theories were made in this time that would provide a catalyst for later scientific breakthroughs.
A book called “Canon of Medicine” by Abu Ali al-Husayn ibn Abd Allah ibn Sina (although known less formally as Avicenna and is one of the greatest early philosopher and fathers of medical science), was the next leap forward. He lived in the early 11th century and wrote one of the earliest treatises on public health. In that work, he comments on the health problems of stagnant water, (6, p787) and how exposure to sun and wind renders such water less harmful. He paid particular note to spring water from high in the mountains and its “sweeter” quality and lower chances of illness developing from consuming it (6, p788). He is the inventor of the concept of distillation, filtration and boiling. His work was not confined to water, however; he commented on the effects of “bad air”, what today we would consider pollution, but framed within the contemporary understanding of miasma - a new disproven theory.
Neither was he alone in the Middle East pushing the yet-to-be-born science forward. Ibn Zuhr, known informally as Avenzoar, discovered scabies mites and hypothesized (quite accurately considering the limits of his time) on their transmission and treatment (7). The first complete description of smallpox is the result of extensive work by another Arab physician, this one called Al-Razi. But Europe was not without its contributions at this early time, however nothing much was achieved until the Renaissance. An early epidemiologist, Girolamo Fracastoro theorized that some diseases could be the result of “seeds” transferring from one body to the other by direct or indirect contact (8) - for example, contaminated clothing.
Empires the world over would continue to use biological warfare. Reports from cities besieged by the Mongols in the 14th-century state that plague victim bodies being catapulted over the walls (5). This was not an isolated incident, nor limited to Mongols, but a continuation of earlier biological warfare.
Enlightenment and the Birth of a Modern Science
The 17th century began as many of the previous had done - with only a rudimentary understanding of microbiology still clinging to outdated beliefs about miasma and hypotheses based on limited evidence that - with the right discovery - could change everything. That would come towards the end of the 16th century (9).
Several key figures would work with it including Galileo, but it had no microbiological application until Englishman named Robert Hooke first used it for this (10). His work was built on by Athanasius Kircher and later by Antonie van Leeuwenhoek (11, 420) who perfected lens design to the extent that it would be useful to physicians. This would provide an important framework heading into the following centuries of Enlightenment. Suddenly, the microbial world was no longer a mystery as van Leeuwenhoek used his new invention to observe bacteria, protozoa, fungi, pollen and spores, and other microorganisms, taken it out of the realm of curiosity and hobbyist.
The 17th century ended up being the age of the microscope; several other key figures came forward in this time such as Malphigi and Swammerdam who observed red blood cells under the microscope (later re-observed by van Leeuwenhoek) (9); he is also famous for conducting the first examination of veins and capillaries as well as cells of organs such as the spleen and kidneys. His work is still important to medical students today. Despite this early success, it would take until 1800 for the first clinical studies to contain information observed in microscopes and it was Marie François Xavier Bichat (12). From there, physicians never looked back.
One of the key discoveries of the late 18th century was Edward Jenner's smallpox vaccination. He was immune from an early age and wanted to understand precisely why survivors of many of the common diseases at the time would not catch them a second time (11, 424). Jenner conducted experiments and successfully demonstrated how infecting a human with cowpox (a near-harmless cousin of smallpox) prevented the patient catching the deadlier disease. However, he did not understand how; it would take some time before anybody figured out why this was the case - he simply figured out the process of preventive measures between similar diseases.
Pasteur and the Golden Age of Microbiology
It is to the great scientists of the age that we look for the next developments in microbiology. Thanks to the microscope and a new understanding that life comes in all shapes, sizes and forms, Louis Pasteur solved the problem for why food - especially dairy - turns sour. His discovery of the presence and processes of bacteria (10) (although he did not discover bacteria - that was van Leeuwenhoek) revolutionized how we think about the harmful microbes in the atmosphere, water and food. He posed the question that if bacteria can make dairy food and wine “ill”, then should it not stand to reason that they can do the same to people? He also posited the idea that they were forms of life and could not have arisen spontaneous - such as miasma theory suggested (12). For millennia, humans had used bacteria in perfectly safe processes such as fermentation to create alcohol, cheese and other dairy produce, and bread (yeast).
Finally, we could begin to understand the biological processes of beneficial and harmful bacteria and the importance of other microbial life. Pasteur is also responsible for the beginnings of synthetic microbial cultivation in 1860. His studies in yeast and sugars were refined later by Ferdinand Cohn and others while the final years of the 19th century saw the invention of the Petri Dish which replaced large bell jars in the cultivation process. It is still used today due to its unique design of slotting together upside down and their lightweight and stackable nature, as well as their cheap production cost (13). The last decade of the 19th century saw the beginning of chemotherapy (11, 425). Although we consider this solely a cancer treatment today, it also has applications in malaria treatment and other conditions too.
Building on the work of Louis Pasteur, Joseph Lister - an English surgeon - effectively created chemical anesthetics in discovering that the types of harmful bacteria that infected patients and could sometimes kill them, could actually be destroyed during the surgery process. Survival rates in hospitals went up at his discovery of the need for cleanliness of operating instruments (14).
The early 20th century continued some of the great inventions and the processes of cultivating microbial life, particularly bacteria. It was also the first time industrial-scale biological warfare was employed. The techniques developed on both sides of the war led to some fundamental scientific discoveries such as the anaerobic jar (11, 423) which developed directly out of the study of the effects of mustard gas. Such stocks supplied on large-scale freed up scientists from having to extract chemicals such as agar in their experiments.
Microbiology in the Modern Age
The next big invention was the electron microscope, arriving in 1940 (10). This allowed for greater resolutions, using a more intense light to create an image of a microorganism, to see even tinier details (15). They came about because physicists realized it was not possible to view organisms smaller than half a micrometer; that proved problematic for most of the microscope's history until that point. Another slightly related invention would also find a use in television - the cathode ray tube or CRT - made it possible to go even deeper. The 1940s is also the decade that virus cultivation exploded (10), leading to an exponential increase in our understanding of viruses which trickled down through the 1950s and 1960s to new vaccines that reduced or eradicated diseases that had killed so many people for centuries. This is the era that also saw the first antibiotics.
But arguably, the discovery of the century was that of DNA - the building blocks of all life demonstrating how all lifeforms are related to each other. We cannot underestimate its importance and we are now only beginning to break through barriers that conventional medicine could previously not break down. Watson & Crick discovered the double helix in 1953 (16), building on the genetics work of Gregor Mendel (17), and it gave birth to modern molecular biology, forensic science, genomics, gene therapy, as well as revolutionizing a wide range of other existing sciences - particularly those using an evolutionary science framework. With its implications for medicine still being realized, this could prove the most important single development in all branches of microbiology.
It hardly seems likely that one of the youngest fields within the science of biology, which got off to a slow start and whose major discoveries and applications came long after the birth of modern science, could permeate our lives so much today. It has applications in medical science as it did at the beginning, but also in ecology and environmental management and remediation - some bacteria are employed to “eat” contaminants and excrete harmless substances. It's used in cosmology as we attempt to understand the biological and chemical processes of our universe, in food science to ensure what we eat is safe (and in researching anthropological and archaeological applications of industrial microbiology such as fermentation). With the opening up of genetics and gene sequencing, we are getting closer to understanding the building blocks of life through the microscope, and not forgetting the positive as well as the negative impact that microbes have on our planet and our lives (10).
The Sub-Disciplines of Microbiology
Each new scientific discovery in any field demonstrates a potential to divide that into separate disciplines and then into subdisciplines as researchers delve deeper into those niche areas. The following is a list of current subdisciplines that exist within microbiology.
Aeromicrobiology
This is the subdiscipline focused on examining minute organisms that survive in the air (18). Microbes exist in all areas of the Earth's ecology and researchers here examine particles that exist, live, thrive and multiply in the air, or diseases that are transmitted through the air. They are known as bioaerosols and can exist in the lower levels of the atmosphere to the highest, in clouds, and can travel great distances through weather systems. The major area of study we might consider is airborne diseases, but this is not the only area - organic atmospheric pollutants, the transmission of pollen and spores for plant reproduction, but also on the chemical processes of how substances crystallize and preserve microorganisms. However, it is also of interest to environmental health inspectors and industry to determine leaks of organic matter and to control and mitigate it.
Agricultural Microbiology
Ever since humanity first began to cultivate crops and livestock for food and other produce, we have sought to understand the physical processes that take place - the effects of genetic modification, the processes of breaking down dead plant material into nutrients, the importance of nutrients for the soil and the chemical and biological processes of algae and other microorganisms in keeping balance in the environment for our agricultural benefit. Agricultural microbiology is the coming together of the wider discipline of microbiology and applying the practical side of biotechnology. The ultimate task is to understand the complex relationship between microorganisms and the environment of agriculture and its processes - including but not limited to: genetics, plant molecular biology, ecological interactions and evolutionary biology. Some argue that agricultural microbiology could prove the next big step in sustainable agriculture by employing microbes to improve yield, longevity, resilience and ecological balance (19) while reducing the traditionally intensive costs of agriculture.
Astromicrobiology
Also known as exomicrobiology, astromicrobiology looks not at our own planet, but to astronomical bodies (20). The vast areas of space are a surprising source of signs of microbiological life, from asteroids that strike the Earth to fossilized remnants of simple life (or chemicals that could have led to life) found on other planets. the main function is to understand how life may start and the conditions under which abiogenesis (creating biological life from inorganic matter) might take place. This process has already been synthesized and now the goal is to identify markers elsewhere in the universe and to search for life no matter how simple or complex. As microbial organic life is the most abundant and the most commonly found in harsh environments, those searching for extraterrestrial life tend to focus their SETI efforts toward life at the micro level, but also on the chemical processes that will lead to liquid water - believed to be necessary for all organic life.
Bacteriology
Bacteriology is the study of bacteria - some of the earliest forms of life to have ever evolved on the planet and still present today (21). We may approach bacteriology from many angles. The most obvious is that of the medical perspective (as some bacteria cause disease), but also from evolutionary microbiology to study how life evolves, from the perspective of industrial application of such useful processes as fermentation to create alcohol, for agriculture such cheese and other dairy products, and the gut flora vital for animal life and humans for digesting food. In each case, bacteria is the tool that permits these processes. Experts in this area may study a wide variety of attributes of bacteria from their genetics to evolution and mutation, their potential medical applications or the diseases they cause (or in some cases, how one bacteria may be used to fight another in a medical application). It has been fundamental in the development of vaccines in the earliest times right through to today and continues to deliver knowledge for disease prevention.
Biotechnology
Biotechnology can come under many of the subdivisions of the biological sciences (22). Within the division of microbiology, it is the application of using microbiology in technological functions and industrial applications. This typically presents us with modern or future applications such as genetic engineering, but arguably it can also be applied to medical biotechnology and the search for organic solutions to the problems of today, environmental remediation (in using microorganisms such as bacteria and viruses to clean up oil spills etc.) However, these future applications are not the only way humans have used microbiology - we have harnessed the natural process of fermentation for millennia - to make bread, cheese and other dairy products, alcohol, and preserving foodstuffs, and as sterilizing agents in simple medical procedures. All of this is biotechnology too.
Cellular Microbiology
Today, biology is a complex and interdisciplinary subject broken down into hundreds of disciplines and subdisciplines. Microbiology is one of them; another is cell biology. It's a relatively young discipline, the term was coined in the mid-1990s and explains the fusing together of these two subdisciplines to fill gaps in the knowledge and explore areas of mutual overlap. Cellular microbiology utilizes microbes in the research of cell biology, and to examine them to explain their pathogenic attributes. However, it is also used to study transmission of biological signals between cells, metabolism, the various cycles and processes of a cell, as well as investigating the biological structure and science of individual cells - rather than simply as a culture. In the future, we expect this area of microbiology to be fundamental in the study of and fight against antibiotic-resistant bugs (23).
Environmental Microbiology
Environmental microbiology is the study of microbes in various environments - water bodies, the air, the soil, in our homes, and their habits in that environment (24). This is a growing area that is expected to subdivide further in the future as it contains so many smaller fields such as microbial ecology, geomicrobiology and microbial diversity (as a form of biodiversity) as well as the other subfields in this list such as aeromicrobiology, to understand the relationships between microbes, between microbes and their environment, and their wider ecological impact - both positive and negative. Here, they study all single and multi-celled organisms that are too small to be seen with the eye including prokaryotes (25), eukaryotes, bacteria and viruses, as well as archaea. Their role cannot be underestimated, either in terms of their ecological impact nor their metabolisms in devouring organic material and the results that come from them. This area has applications in environmental remediation, for altering an ecology for human habitation - which is expected in future to include terraforming other planets.
Evolutionary Microbiology
This small niche area has and will continue to prove vital in the study of evolutionary biology (26). As already discussed, microbes represent the earliest forms of life on our planet. Understanding how they evolve and the development of DNA, proteins and other simple chemical and biological processes could be the key to unlocking all of life. Specialists in this area could work on a number of subareas Including microbial taxonomy (defining microbial life into categories and subcategories, the naming and classification) and explain the various evolutionary relationships, mutations, and environmental forcings on such microbes, and microbial systematic which is the study of genetic relationships between the microbes and of diversity.
Food Microbiology
This area examines many issues related to our food. Our relationship with food microbes is very mixed - some positive (creating or transforming foodstuffs) and some negative (infecting and contaminating) while others are just present as a natural byproduct of food processing, such as yogurt (inhabiting). It can cover food production safety issues (27) such as the presence of illness in livestock, bacteria in food handling due to it passing safety levels, the processes and cleanliness of the production facilities, but also examining the natural and artificial biochemical processes that turn food from one state to another. We utilize bacteria safely in the production of dairy produce, alcohol and breadmaking. Some of these have been vital to human civilization's development over thousands of years. However, it will also look at industrial microbiology, using algae and other microorganisms as thickening agents
Generational Microbiology
This small niche subdiscipline of microbiology examines the issues surrounding heredity - this is possible in the simple single cell organisms. It could help to shed light on some of the issues surrounding the evolution from simple to complex life forms. To understand how and why genetic mutation occurs and its role in shaping a genetic makeup could be key to understanding all life.
Immunology
This is the branch of medical science that studies the role and function of the immune system in any living organism. Part of this study in humans and in other animals is examining the role of microorganisms on a biological body (28), both as invaders that would do the host body harm and as “defensive” bacteria in the body that helps to keep the biological entity healthy. It is also concerned with microbes in the immune system that concern raising a “false flag” resulting in allergies. The relationship between complex lifeforms and microbes has always been one of symbiosis in terms of the positive relationships and one of battle with those deemed negative. Immunology as a branch of microbiology seeks to improve longevity and quality of life through vaccines and other preventive measures, medicines and other aftercare treatment. More recently, researchers have been studying the genetic codes of microbes to understand how we can better combat their causes at the microbial level.
Industrial Microbiology
This is the human application of microbes for commercial or industrial processes, some of which we have already discussed. Typically, it involved the cultivation and processing of microbes to produce many of our most common foods such as fermentation for alcohol production, yeast fermentation to produce bread, the processes that lead to making cheese and other dairy products, but on an industrial scale. Also, this includes the cultivation of bacteria that create yogurt. These are some of the oldest ways in which we harness the power of microbes in the environment. More modern processes involved identifying algae and bacteria useful in water waste treatment. Some “eat” toxic substances and render them inert or produce harmless byproducts because of that consumption. Also, we are now employing microbiology in medicine and vitamin production to cope with growing demand of a growing population (29).
Microbial Studies
Microbial studies is more concerned with the physical structure and function of a microbe than its processes. Largely, this area is broken down into four general groups. These are microbial cytology which is the study of minute details on a microbe; microbial physiology which examines cell functionality; microbial ecology which is the examination of their relationship within their environment; and microbial genetics which is the examination of a microbe's genetic structure.
Military Microbiology / Microbiological Agents
As discussed in an earlier section, humans have used microbiology as a weapon for millennia - fungi, viruses, bacteria, algae, and other toxic microorganisms (30). Initially, this was crude and involving sending raw materials infected with harmful agents against enemies, for example, blankets and clothing that were worn by people who died from plague, cholera or smallpox. It also involved using catapults to send infected bodies over walls of cities under siege. In more recent times, it concerns the use of biological agents in war and in terrorism, starting with WWI and the use of chemical and biological warfare using botulism, ricin, anthrax and others. This was outlawed internationally in 1972 but research continues largely for two reasons - to examine strains of such diseases to determine their effects and to develop vaccines, treatments, or cures, and research and prevention for the purposes of building international evidence for intervention against regimes that use them.
Molecular Microbiology
Providing a bridge between biochemistry and microbiology, molecular microbiology studies the molecular causes for why biological entities and their cells act in the way they do (31). Typically, they will look at interactions between RNA and DNA, in proteins, and all other biochemical systems. It's considered an approach rather than a technique, looking at biological systems functionality and encouraging researchers in other areas of biology to think about the molecular framework of the biological system rather than only at the macro level. As advances in genetics progress, this area is expected to prove vital in our understanding of all biological sciences, not just for microbiology.
Mycology
This is the study of fungi and their biological processes. It was once assumed that the humble fungus was a plant; however, their genetic structure and other biological indicators suggest they are in a class of their own, neither animal nor plant, and - most surprisingly - more closely related to all animals than to any plant. Mycologists examine all things that a botanist might study for plants but for fungi instead such as genetics, reproduction, evolution, taxonomy, habitat, ecology, disease pathology, and so on. Mycology is useful in a number of areas, most notably in evolutionary biology due to the vital ecological role than they play. They can occur as a yeast or a mold; some cause diseases and others have antiseptic or antibiotic properties from which we derive such medicines.
Nanomicrobiology
It is possible to look at aspects of a biological entity at a much smaller scale than the cellular. Nanomicrobiology studies the aspects within the cell (32). This includes the form and function of a cell wall and everything inside the cell. The importance of this area of study cannot be underestimated. It is in the cell wall that we understand and explain how a virus might mutate - this is useful for planning vaccines in any given influenza season. More recent advances in microscopes have allowed us to look even deeper than ever before, to examine microbe cell surfaces and to correct many of the misunderstandings we had about cells before we had the ability to examine them on the nano level. Some of these questions include how morphology might change with chemical interaction (uses of medical drugs), and what drives antibiotics to neutralize the effects of such cells.
Nematology
Nematodes (also known as roundworms) are a type of parasitic worm too small to be seen with the naked eye. Their study is referred to as “nematology” (33). Until the invention of the microscope, it was not possible to study these. The biological science of nematology is just 150 years old even though interest in microscopic parasites go back thousands of years when civilizations understood the effects they could have but without the ability to see them. Nematologists examine everything you can expect any other biological specialist to research - feeding, life cycle, reproduction, genetics, ecology, environments, and of course the diseases they can cause in animals and humans. Also, some may examine them from a medical perspective in studying their treatment. Some are not outright harmful to humans but can infect our most important food crops, and causing crop failure and famine.
Parasitology
While nematology studies parasitic worms, parasitology as a subdiscipline of microbiology studies all minute parasites. These lifeforms are a group of biological entities that can only survive on or in another lifeform (34). Parasites exist in the animal and plant kingdom. In most cases, a parasite harms the host body. This is different from symbiotic relationships where there is a mutual benefit. Some argue that modern parasitology also studies (or should study) instances of symbiotic relationships. This area of study has many applications. We might consider it typically associated with human diseases such as ringworms and flatworms, it also concerns parasitology as problems for agriculture. One of the biggest issues today is the effect of the varroa mite on honeybee populations globally. Evidence suggests that the growth in instances of this parasite is a major contributing factor to CCD (35).
Pharmaceutical Microbiology
How do the microbiological entities present in our medicines work? What in their profile causes them to have the effects that they have? Pharmaceutical microbiology is the study of microorganisms and their relationship to pharmaceutical drugs. They are concerned with the questions above but also with safety and research integrity, compliance, health issues, and quality assurance and quality control (36), such as ensuring equipment is sterile during processing and removing the byproducts of microbes from water and other organic or chemical materials used in research. This is also a useful discipline in detecting potential side effects such as carcinogenicity, infection control, mutagenic issues and using microorganisms to alter the chemical state of a product - examples include human growth hormones and insulin.
Phycology
The study of algae has its own separate discipline within botany - (37). However, it also comes under microbiology because despite being able to see the physical presence of algae on water, it is impossible to see the structure of these minute lifeforms without a microscope. They are tiny microbes, often eukaryotic (single-celled) and vital to aquatic ecosystems the world over. They represent some of the earliest forms of life, differing from other plants in that they lack roots, stems, flowers and most other signs of being plants. Phycology also covers plankton and seaweed such as kelp, and multi-celled organisms such as the blue-green algae and cyanobacteria, and lichen. Ancient cultures knew about these lifeforms and some even cultivated them for agricultural and medicinal use. Modern uses of algae include environmental remediation as some devour pollutants and create harmless byproducts.
Phylogenetics
Many of the subdisciplines (and microbiology as an umbrella term) study biological entities on the cellular level in isolation (38). But phylogenetics looks at the evolutionary history of species, and evolutionary relationships, at the cellular level. Researchers do this by comparing genes and proteins between biological organisms to determine where on the family tree of life they may have diverged and for identifying potential common ancestors in paleontological sequences. Once useful only in biology, a “family tree” of life can help to piece together biological sequences at the microscopic level for purposes of taxonomy. Today, we can use such evolutionary trees for all forms of life - simple and complex, for tracing pathogens and their genetic mutations, and searching for common ancestors for most species. Using genetic studies as well as assemblage profiles, the tree may be complete by looking at the cellular level.
Predictive Microbiology
This is the application of technology such as predictive modelling in determining how a microorganism might react in any environment, under any given circumstances, and what factors might influence a change in behavior. Being able to predict what a microorganism might do or how it might react has many complex uses but remains a theoretical framework at present as not all factors can always be accounted for (39). The most obvious and prevalent application would be predicting how viruses might spread or mutate and what factors may be useful in its eventual decline. Predictive microbiology assumes that the actions and results of microorganisms are predictable and reproducible based on such factors as genetics and past history. Other uses include environmental and food safety science, looking at safe and unsafe levels of bacteria, the actions of harmless bacteria and other inclusions and what might happen to make them unsafe.
Soil Microbiology
With many uses in agriculture for improving yield and resilience - especially where problems of long-term monoculture are concerned (40), ecology for environmental balance, land management, and environmental remediation, soil microbiology is an important niche area that studies the microbes in soils to understand the process of how they break down dead organic material to produce nutrients. It is largely through these microbes that vegetation will break and digest down these nutrients in order to sustain growth. Microbes are a vital part of the soil nutrient cycle and that is no better demonstrated than in soils.
Protistology
Microscopic single cell organisms do not have a single profile. Some are plant-like while others share many more attributes with animals than they do with bacteria (independent movement, feeding such as predation) or algae and other plants (41). It is this animal-like group that interests protistology - also known as protozoology. This group of single-cell organisms is known as “protozoa” and people who work in this area seek to understand everything about their biology that a typical zoologist seeks to understand. This can include parasites and symbiotes as well as predator and prey behavior. There is much debate over classification and taxonomy because referring to them as “single cell animals” is wrong even though they display some attributes more closely related to animal than plant behavior. Typical examples of lifeforms that protistologists might study include the parasite that causes malaria and those that digest harmful microfungi and bacteria in the environment.
Systems Microbiology
This is more of an application than a subdiscipline but using cross-disciplinary information to create a profile of a specific microbe to determine its nature, function and behavior. It uses information from areas such as genetics and genomics, metabolism, proteomics and many more to attempt to compile such a picture (42). Its useful in many areas, most notably in examining how bacteria become resistant to antibiotics, looking at the processes of metabolism in digestion, and disease transmission.
Veterinary Microbiology
Experts who work in this area examine microbiology (viruses, bacteria, fungi, algae) from a zoological perspective (43) - that is, vertebrate animals rather than in humans or in terms of their impact on the environment. They will examine health and disease, digestion, symbiotic and parasitic relationships, agriculture and the environment. As with other areas, sometimes these microbes are harmful and sometimes they are harmless. In other cases, we may find that certain animals have a natural immunity to some bacteria or related conditions that can reach epidemic or endemic proportions in humans. We may study this to understand precisely why such animals have immunity.
Virology
Microbes come in many taxa and this is the branch of microbiology which examines viruses. These are parasitic microbes that can only spread and reproduce within a host body. A microbe is identified as a virus if it has a coat of protein inside which is contained genetic data. It is one of the simplest forms of life, one of the most prevalent and most potentially dangerous for life due to the effects that they have when infected a host. Virologists examine the nature of transmission, their genetic code, pathology, mutation and every other aspect related to a virus. Much of medical history has been dedicated to fighting against viruses and its spread; however, there are many positive uses. As discussed above, viruses have always had fundamental uses in the vaccination process. Today, in some cases, we are using viruses to deliver treatments in the fight against other diseases (44). Viruses may yet be the determining factor in future cancer treatments.
Water Microbiology
We have already covered aeromicrobiology (microbes in the air) and soil microbiology (microbes in soils) - this examines everything to do with microbes in the water (45). This covers bacteria, fungi, viruses, microparasites, protists and everything else. It is sometimes called marine microbiology when studied as a subdiscipline of environmental microbiology. Such microbes can be beneficial (eating pollutants to keep the water fresh, clean and safe for other life) or harmful (water borne diseases). Yeast is one example of a useful water-borne microbe, it ferments to create bread, while the highly dangerous bacteria called E. coli is a major cause of food poisoning.
Current and Future Challenges and Trends for Microbiology
Antibiotic-Resistant Bacteria
Antibiotics are used to combat bacterial infection. However, their overuse in recent years, driven partly by pressure from patients and partly by doctors having an increasingly limited amount of time and information to make a diagnosis playing it safe and prescribing antibiotics when the patient may have a virus, means that our bodies are increasingly becoming immune to the effects of this vital and important medication. Increasingly, researchers are being forced to look elsewhere for new antibiotics to fight back against antibiotic resistance and superbugs on which they have no effect. Recent studies have revealed new potential treatments in soil (46) and another in the human nasal passage (47). In 2014, the United Nations declared antibiotic resistance the next global health crisis.
Bioterrorism
As stated above, biological warfare was outlawed in the 1970s. However, the threat of bioterrorism and biological attack has never gone away due to the ongoing presence of rogue states and terrorist organizations. Warfare has fundamentally changed in the 21st century. All-out war is less likely now but what is more likely is organized terrorist attack by small cells on key targets. Biological agents such as botulism and anthrax can cause massive loss of human life and long-term health difficulties. It is to clinical microbiologists (52) that we seek answers in terms of identification, building evidence in prosecution of individuals, reporting to international bodies in cases for sanctions or military action against rogue states, and of course, for vaccines and treatments. Bioterrorist actions could potentially be directed at humans, at livestock and the food supply, water supplies and dispersed aerially for maximum infection.
Disease Eradication
This has always been a challenge for medical scientists; smallpox eradication in the 1950s-60s is one of medical science's and public health's greatest accomplishments. But many more diseases remain virulent. It took decades and over $2bn to eradicate polio from India. The challenge to do something about malaria and tuberculosis is expected to be just as long, just as hard, and just as expensive. Recent developments in genetics show several potential new pathways in such treatments. Researchers have conducted trials that successfully used bacteria to kill the parasite in mosquitoes that cause malaria (50) without killing the mosquito (which fills a vital ecological function). Of all places to find this bacteria, it was never expected that mosquitoes carry it in their stomach. Similar tactics were used against the Zika outbreak in Central America in 2016-17.
Food Poisoning as a Long-Term Public Health Issue
This is nothing new; after all, we now all know the potential short-term dangers of E. coli and salmonella. However, the age of industrialized agriculture is starting to reveal other potential problems of food poisoning - that of long-term health. This issue is still largely misunderstood. Food poisoning has the potential to kill, but it can also cause long-term health effects and recurring problems, especially when the cause of an infection results in permanent scarring or changes to bodily functions. Also, we expect further potential problems of food poisoning as climate changes and alterations to an agricultural ecology leads to the growth of bacteria that leads to food poisoning (48, p60).
Microbiomes
All biological sciences in recent decades have moved away from studying lifeforms in isolation to a more holistic approach, or studying the ecologies of those lifeforms. This is the next step for microbiology as we begin to understand the genetics of microbes and their importance to all environments. It's still a small movement within microbiology, but we expect rapid growth in the study of so-called “microbiomes”. This is the ecology of microbe cultures and their relationship to each other. So far, most understanding has come from microbes of the human digestive system (49), but it is expected to branch out with more research in the coming decade. Its advent comes as the result of new gene sequencing methods and the ability to acquire, store, process and handle big data.
Sustainability
Our population is continually growing. As urban development and industrial zones continue to encroach into new lands, there is less land for agriculture. But we need more agricultural land to feed the growing population. It is likely that researchers in microbiology will play a fundamental part in not just improving yield for a greater population, but also to ensure that our key crops - food, raw materials such as vegetable oils and cotton, and biofuel production, are produced sustainably. It is vital that while improving economic well being, the environments in which such crops grow is not damaged through monoculture and a lack of biodiversity. Many of these exist in nature and provide a vital ecological function. In future, we expect more microbes (either naturally occurring or genetically modified) microbes to fulfill the same functions (51).
Learn more about the subject of sustainability and the green careers associated.
Sources
- https://microbiologysociety.org/about/what-is-microbiology-.html
- https://microbiologysociety.org/why-microbiology-matters/what-is-microbiology/microbes-and-the-human-body/vaccination.html
- http://digitalcommons.lmu.edu/cgi/viewcontent.cgi?article=1001&context=theo_fac
- https://www.sciencedirect.com/science/article/pii/S1198743X14641744
- https://pdfs.semanticscholar.org/9818/7d6c789ddd1396be9ae810a2cb279ab7b709.pdf
- https://www.ncbi.nlm.nih.gov/pmc/articles/PMC1865595/
- http://infectiousdiseases.edwardworthlibrary.ie/theory-of-contagion/
- http://www.annclinlabsci.org/content/32/3/309.full
- https://www.cliffsnotes.com/study-guides/biology/microbiology/introduction-to-microbiology/a-brief-history-of-microbiology
- https://pdfs.semanticscholar.org/792e/b385b8a99f88cee61e302e2d6048d922b8d3.pdf
- https://www.biodiversitylibrary.org/item/210666#page/313/mode/1up
- https://profiles.nlm.nih.gov/SC/Views/Exhibit/narrative/doublehelix.html
- https://link.springer.com/chapter/10.1007%2F978-94-009-2057-6_5
- https://onlinelibrary.wiley.com/doi/abs/10.1111/j.1744-7348.2011.00489.x
- https://www.ncbi.nlm.nih.gov/books/NBK8120/
- https://www.bio.org/what-biotechnology
- https://www.ncbi.nlm.nih.gov/pmc/articles/PMC139013/
- https://www.ncbi.nlm.nih.gov/pubmed/20670913
- https://www.hindawi.com/journals/ijmicro/2012/863945/
- https://www.immunology.org/public-information/what-immunology
- https://www.ncbi.nlm.nih.gov/pubmed/1368195
- https://www.osha.gov/SLTC/biologicalagents/index.html
- https://www.nature.com/articles/1901124a0
- https://www.ncbi.nlm.nih.gov/pmc/articles/PMC3246382/
- https://www.sciencedirect.com/topics/agricultural-and-biological-sciences/nematology
- https://www.path.cam.ac.uk/directory/research-themes/parasitology
- https://www.sciencedirect.com/science/book/9780081000229
- https://www.britannica.com/science/phycology
- https://www.sciencedirect.com/topics/neuroscience/phylogenetics
- https://www.ncbi.nlm.nih.gov/pubmed/7873329
- https://www.nature.com/articles/s41598-018-24537-2
- https://books.google.co.uk/books?id=sYgKY6zz20YC&pg=PA130&lpg=PA130&dq=panno+the+cell&redir_esc=y&hl=en#v=onepage&q=panno%20the%20cell&f=false
- http://www.ux.uis.no/~ruoff/systemsmicrobiology_ch08.pdf
- https://www.journals.elsevier.com/veterinary-microbiology/
- http://science.jrank.org/pages/7311/Water-Microbiology.html
- https://www.the-scientist.com/?articles.view/articleNo/41850/title/New-Antibiotic-from-Soil-Bacteria/
- http://www.sciencemag.org/news/2016/07/new-antibiotic-found-human-nose
- https://pdfs.semanticscholar.org/0ede/1703bfd3351e31eb6d81ebe7306842edd27d.pdf
- https://www.ncbi.nlm.nih.gov/pmc/articles/PMC3426293/
- https://www.sciencedaily.com/releases/2011/05/110512150729.htm
- https://www.sciencedirect.com/science/article/pii/S0167779916300841
- http://cmr.asm.org/content/14/2/364.full
- Guide to Parasitology - November 19, 2018
- Deserts as Ecosystems and Why They Need Protecting - November 19, 2018
- Conservation: History and Future - September 14, 2018